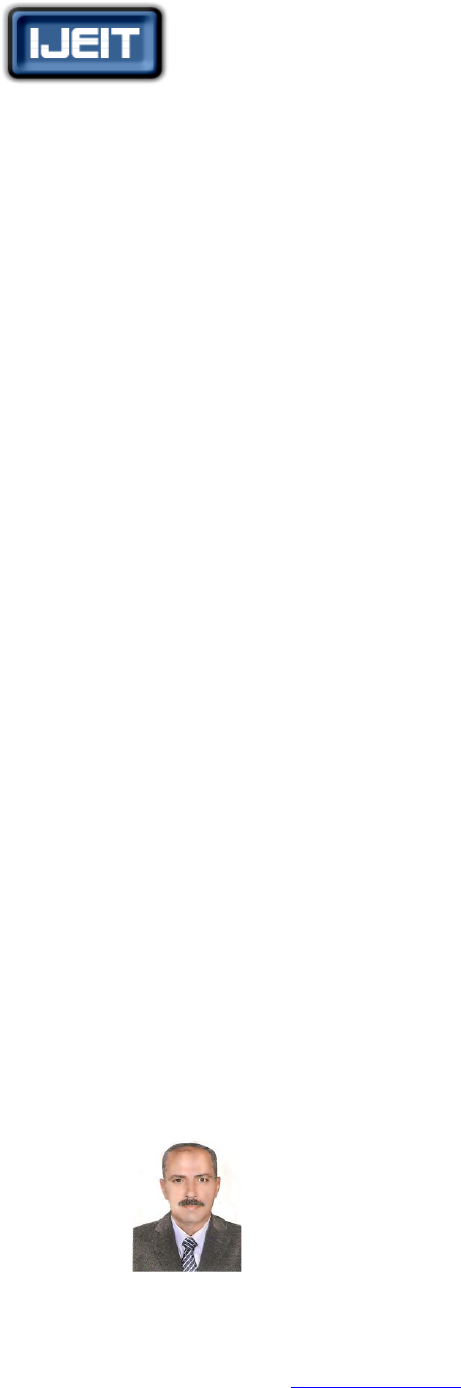
ISSN: 2277-3754
ISO 9001:2008 Certified
International Journal of Engineering and Innovative Technology (IJEIT)
Volume 3, Issue 6, December 2013
215
The figures indicate the following:
All grains of the deformed samples were elongated in the
direction of cold rolling. This elongation is not affected by
the chemical machining process.
Average of measured surface roughness of the machined
samples without cold working is 2 µm, which is too close
to that can be calculated by the designed empirical model
where Ra =2.5µm and MRR=0.01 g/min. The average
surface roughness for machined samples with 20% cold
working was 1.68µm, which is also close to that can be
calculated by the designed empirical model, where Ra =
2µm and MRR=0.01 g/min. This indicates the confidence
of the designed empirical models.
There are no metallurgical defects on the machined
surfaces due to chemical machining process.
Microstructure is consisting of two phases (γ + M), dark
and light color, austenite phase (γ) and martensite phase
(M). After chemical machining, there is no change in
microstructure, but an increase in martesite phase (M) had
been occurred. For samples with 20% cold working the
microstructure before chemical machining contains
elongated martensite (M) within matrix of austenite phase
(γ) in crystal lattice, as the transformation of the induced
plasticity causes the grain size to be decreased.
VIII. CONCLUSIONS
Based on the detailed results the following conclusions can
be stated:
1. Machining time, machining temperature and previous
cold working are important variables that affect on
chemical machining products; among these variables
machining temperature has the largest effect.
2. Surface roughness of chemically machined parts
increases with the machining temperature and machining
time.
3. Surface roughness of chemically machined parts
decreases with the previous cold working of the work
piece.
4. Metal removal rate increases with machining
temperature and decreases with previous cold working.
5. Products of stainless steel 420 without cold working can
be chemically machined in [H
2
O + HCl + HNO
3
+ HF +
HCOOH] etchants in optimum conditions at temperature
45ºC and time 6 min.
6. Products of stainless steel 420 with 20% cold working
can be chemically machined in [H
2
O + HCl + HNO
3
+
HF + HCOOH] etchants in optimum conditions at
temperature 40 ºC and time 6 min.
7. An assessment of CHM can be achieved by empirical
modelsfor selecting the appropriate machining
conditions for the required surface roughness and metal
removal rate.
REFERENCES
[1] Abu Khalid Rivai, 2012, "Effect of Cold Working on The
Corrosion Resistance of JPCA Stainless Steel in Flowing"Pb–
Bi at 450◦C, Journal of Nuclear Materials 431, PP (97–104).
[2] Benedict.G.F, 1987, "Nontraditional Manufacturing
Processes", Mercel Decker Inc., New York, USA.
[3] Blak.JT, DeGarmo, 2007, "Materials and Processes in
Manufacturing", John Wiley & Sons, Inc, Tenth ed.
[4] David M. Allen, 2004, "Characterization of Aqueous Ferric
Chloride Etchant Used in Industrial Photochemical
Machining", School of Industrial and Manufacturing Science,
Cranfield University, UK.
[5] Drozda.T.J, 1989, "Tool and Manufacturing Engineers",
Handbook (Chapter 14: Nontraditional Machining), SME
Publishing.
[6] El-Hofy.H.A.-G, 2005, "Advanced Machining Processes",
Black lick, OH, USA, McGraw-Hill Companies.
[7] FadaeiTehrani.A, 2004, "A New Etchant For The Chemical
Machining of St304", Journal of Materials Processing
Technology 149, PP (404–408), Isfahan University of
Technology, Isfahan, Iran.
[8] Ho S., 2008, "Chemical Machining of Nanocrystalline Ni",
Department of Materials Science and Engineering, University
of Toronto, 184 College Street, Toronto, Canada.
[9] Kurc A. , 2010, "Influence of Cold Rolling on The Corrosion
Resistance of Austenitic Steel", Journal of Achievements in
Materials and Manufacturing Engineering, Volume 38 Issue 2.
[10] Langworthy M., 1994, "Chemical Milling By Nontraditional
machining process", Machining ASM Handbook.
[11] Philip A. Schweitzer, 2010, "Fundamentals of Corrosion,
Mechanisms", Causes and Preventative Methods, CRC Press
© by Taylor and Francis Group, LLC
[12] SeropeKalpakjian, 2006, "Manufacturing, Engineering &
Technology, Fifth Edition", Pearson Education, Inc., Upper
Saddle River, NJ.
[13] SumanSamanta, 2011, "Parametric Optimization of Some
Non-traditional Machining Processes Using Artificial Bee
Colony Algorithm, Department of Production Engineering",
Jadavpur University, Kolkata, India.
[14] Yao Fu, 2009, "Effects of Cold Work and Sensitization
Treatment on The Corrosion Resistance of High Nitrogen
Stainless Steel in Chloride Solutions", ElectrochimicaActa 54,
PP (1618–1629).
AUTHOR BIOGRAPHY
Prof. Dr. Haydar A.H. Alethari
Department of Materials Engineering,
Babylon University,
Hilla-Najaf Road, P.O. 4- Al-Hilla, Iraq
E-amail: draletharihah@yahoo.com
Dr.Eng.Alethari@uobabylon.edu.iq
Haydar A.H. Alethari obtained his BSc. in Mechanical
Engineering from Mosul University-Iraq in 1981.
Then, he obtained his MS and PhD degrees from
Kharkov University (USSR-Ukraine) in 1988 and
1991, respectively. He is interested and a researcher in manufacturing,
traditional and advanced cutting processes of composites and metals.
Currently, he is a team leader of MSc. students in Materials Engineering,