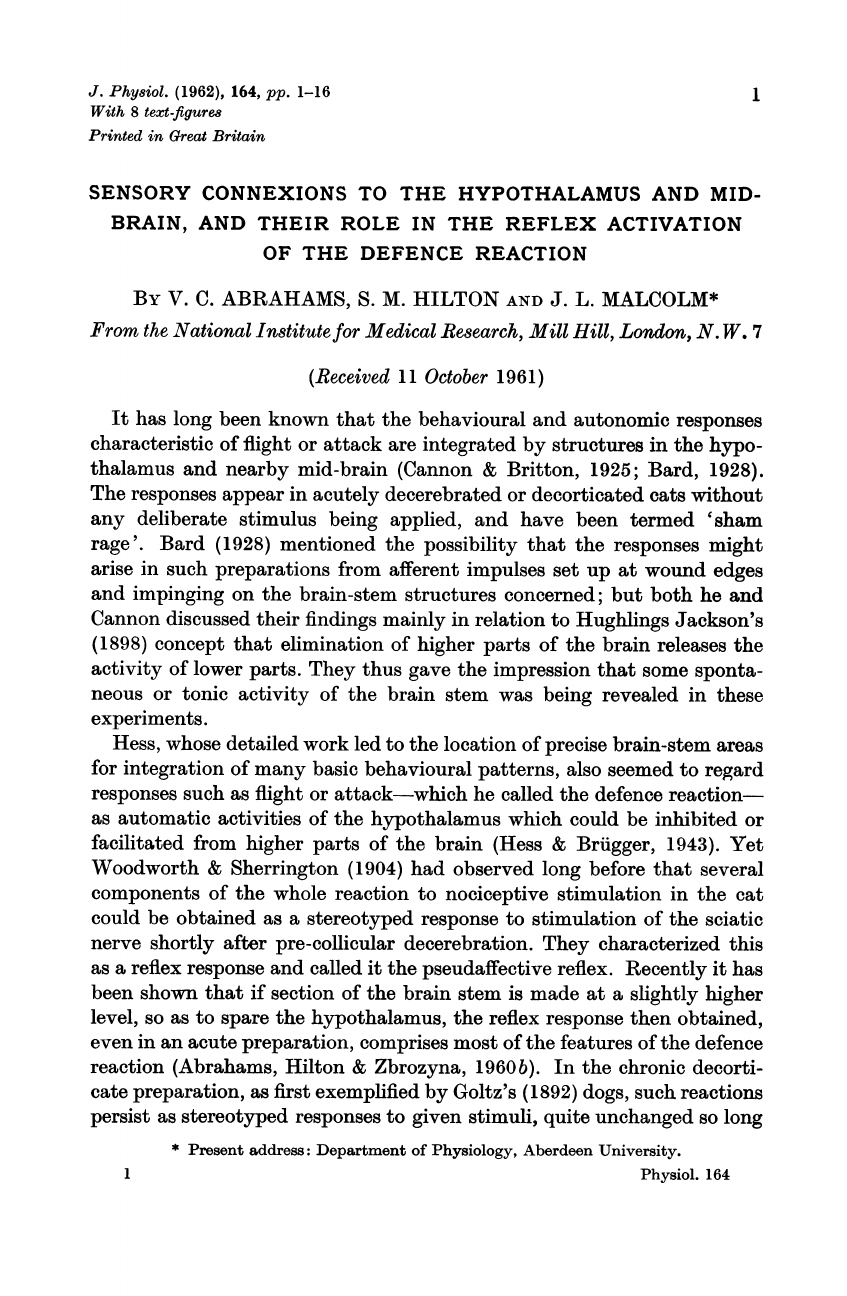
J.
Phya8ol.
(1962),
164,
pp.
1-16
1
With
8
text-figure8
Printed
in
Great
Britain
SENSORY
CONNEXIONS
TO
THE
HYPOTHALAMUS
AND
MID-
BRAIN,
AND
THEIR
ROLE
IN
THE
REFLEX
ACTIVATION
OF
THE
DEFENCE
REACTION
BY
V.
C.
ABRAHAMS,
S.
M.
HILTON
AND
J.
L.
MALCOLM*
From
the
National
Institute
for
Medical
Research,
Mill
Hill,
London,
N.
W.
7
(Received
11
October
1961)
It
has
long
been
known
that
the
behavioural
and
autonomic
responses
characteristic
of
flight
or
attack
are
integrated
by
structures
in
the
hypo-
thalamus
and
nearby
mid-brain
(Cannon
&
Britton,
1925;
Bard,
1928).
The
responses
appear
in
acutely
decerebrated
or
decorticated
cats
without
any
deliberate
stimulus
being
applied,
and
have
been
termed
'sham
rage'.
Bard
(1928)
mentioned
the
possibility
that
the
responses
might
arise
in
such
preparations
from
afferent
impulses
set
up
at
wound
edges
and
impinging
on
the
brain-stem
structures
concerned;
but
both
he
and
Cannon
discussed
their
findings
mainly
in
relation
to
Hughlings
Jackson's
(1898)
concept
that
elimination
of
higher
parts
of
the
brain
releases
the
activity
of
lower
parts.
They
thus
gave
the
impression
that
some
sponta-
neous
or
tonic
activity
of
the
brain
stem
was
being
revealed
in
these
experiments.
Hess,
whose
detailed
work
led
to
the
location
of
precise
brain-stem
areas
for
integration
of
many
basic
behavioural
patterns,
also
seemed
to
regard
responses
such
as
flight
or
attack-which
he
called
the
defence
reaction-
as
automatic
activities
of
the
hypothalamus
which
could
be
inhibited
or
facilitated
from
higher
parts
of
the
brain
(Hess
&
Briigger,
1943).
Yet
Woodworth
&
Sherrington
(1904)
had
observed
long
before
that
several
components
of
the
whole
reaction
to
nociceptive
stimulation
in
the
cat
could
be
obtained
as
a
stereotyped
response
to
stimulation
of
the
sciatic
nerve
shortly
after
pre-collicular
decerebration.
They
characterized
this
as
a
reflex
response
and
called
it
the
pseudaffective
reflex.
Recently
it
has
been
shown
that
if
section
of
the
brain
stem
is
made
at
a
slightly
higher
level,
so
as
to
spare
the
hypothalamus,
the
reflex
response
then
obtained,
even
in
an
acute
preparation,
comprises
most
of
the
features
of
the
defence
reaction
(Abrahams,
Hilton
&
Zbrozyna,
1960b).
In
the
chronic
decorti-
cate
preparation,
as
first
exemplified
by
Goltz's
(1892)
dogs,
such
reactions
persist
as
stereotyped
responses
to
given
stimuli,
quite
unchanged
so
long
*
Present
address:
Department
of
Physiology,
Aberdeen
University.
1
Physiol.
164
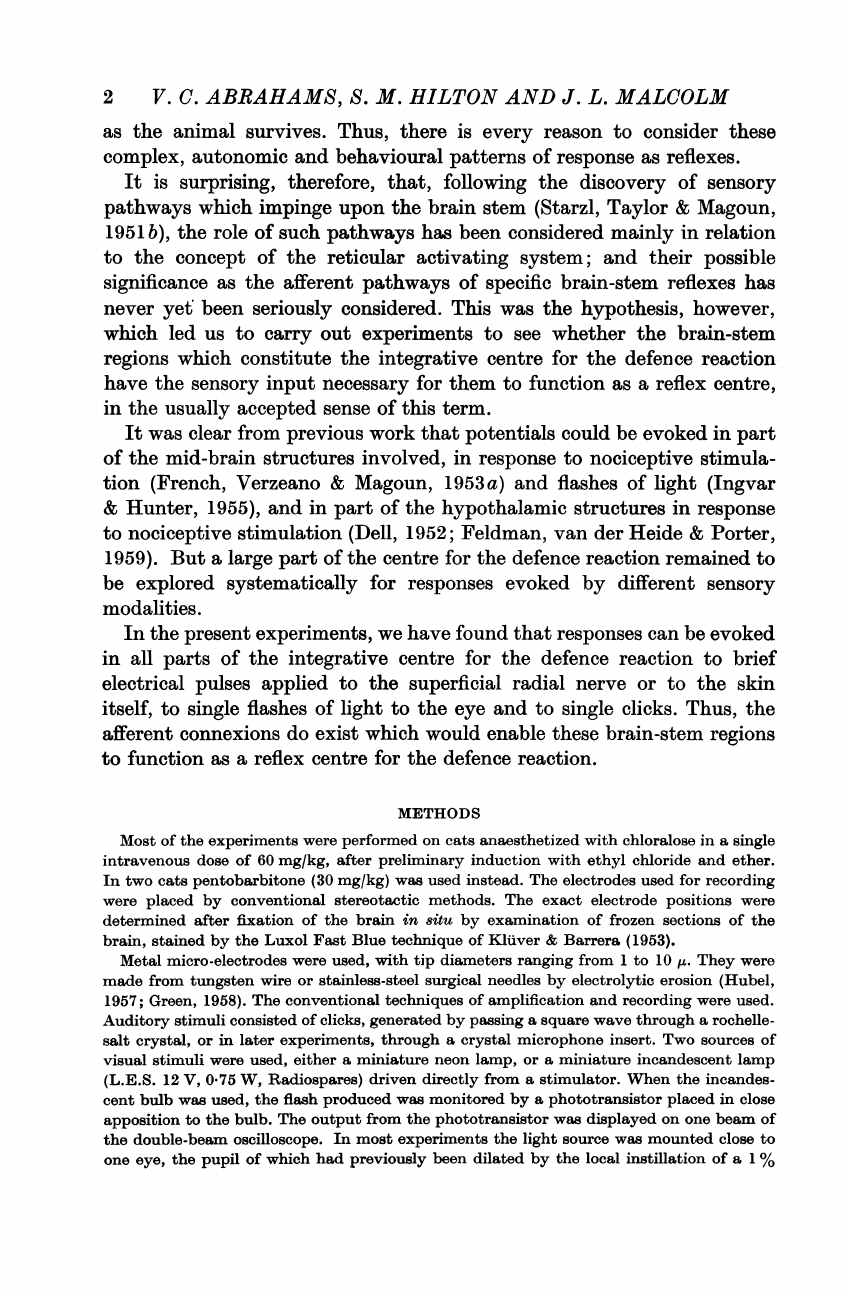
2
V.
C.
ABRAHAMS,
S.
M.
HILTON
AND
J.
L.
MALCOLM
as
the
animal
survives.
Thus,
there
is
every
reason
to
consider
these
complex,
autonomic
and
behavioural
patterns
of
response
as
reflexes.
It
is
surprising,
therefore,
that,
following
the
discovery
of
sensory
pathways
which
impinge
upon
the
brain
stem
(Starzl,
Taylor
&
Magoun,
1951
b),
the
role
of
such
pathways
has
been
considered
mainly
in
relation
to
the
concept
of
the
reticular
activating
system;
and
their
possible
significance
as
the
afferent
pathways
of
specific
brain-stem
reflexes
has
never
yet
been
seriously
considered.
This
was
the
hypothesis,
however,
which
led
us
to
carry
out
experiments
to
see
whether
the
brain-stem
regions
which
constitute
the
integrative
centre
for
the
defence
reaction
have
the
sensory
input
necessary
for
them
to
function
as
a
reflex
centre,
in
the
usually
accepted
sense
of
this
term.
It
was
clear
from
previous
work
that
potentials
could
be
evoked
in
part
of
the
mid-brain
structures
involved,
in
response
to
nociceptive
stimula-
tion
(French,
Verzeano
&
Magoun,
1953
a)
and
flashes
of
light
(Ingvar
&
Hunter,
1955),
and
in
part
of
the
hypothalamic
structures
in
response
to
nociceptive
stimulation
(Dell,
1952;
Feldman,
van
der
Heide
&
Porter,
1959).
But
a
large
part
of
the
centre
for
the
defence
reaction
remained
to
be
explored
systematically
for
responses
evoked
by
different
sensory
modalities.
In
the
present
experiments,
we
have
found
that
responses
can
be
evoked
in
all
parts
of
the
integrative
centre
for
the
defence
reaction
to
brief
electrical
pulses
applied
to
the
superficial
radial
nerve
or
to
the
skin
itself,
to
single
flashes
of
light
to
the
eye
and
to
single
clicks.
Thus,
the
afferent
connexions
do
exist
which
would
enable
these
brain-stem
regions
to
function
as
a
reflex
centre
for
the
defence
reaction.
METHODS
Most
of
the
experiments
were
performed
on
cats
anaesthetized
with
chloralose
in
a
single
intravenous
dose
of
60
mg/kg,
after
preliminary
induction
with
ethyl
chloride
and
ether.
In
two
cats
pentobarbitone
(30
mg/kg)
was
used
instead.
The
electrodes
used
for
recording
were
placed
by
conventional
stereotactic
methods.
The
exact
electrode
positions
were
determined
after
fixation
of
the
brain
in
8itu
by
examination
of
frozen
sections
of
the
brain,
stained
by
the
Luxol
Fast
Blue
technique
of
Kluver
&
Barrera
(1953).
Metal
micro-electrodes
were
used,
with
tip
diameters
ranging
from
1
to
10
g.
They
were
made
from
tungsten
wire
or
stainless-steel
surgical
needles
by
electrolytic
erosion
(Hubel,
1957;
Green,
1958).
The
conventional
techniques
of
amplification
and
recording
were
used.
Auditory
stimuli
consisted
of
clicks,
generated
by
passing
a
square
wave
through
a
rochelle-
salt
crystal,
or
in
later
experiments,
through
a
crystal
microphone
insert.
Two
sources
of
visual
stimuli
were
used,
either
a
miniature
neon
lamp,
or
a
miniature
incandescent
lamp
(L.E.S.
12
V,
0-75
W,
Radiospares)
driven
directly
from
a
stimulator.
When
the
incandes-
cent
bulb
was
used,
the
flash
produced
was
monitored
by
a
phototransistor
placed
in
close
apposition
to
the
bulb.
The
output
from
the
phototransistor
was
displayed
on
one
beam
of
the
double-beam
oscilloscope.
In
most
experiments
the
light
source
was
mounted
close
to
one
eye,
the
pupil
of
which
had
previously
been
dilated
by
the
local
instillation
of
a
1
%
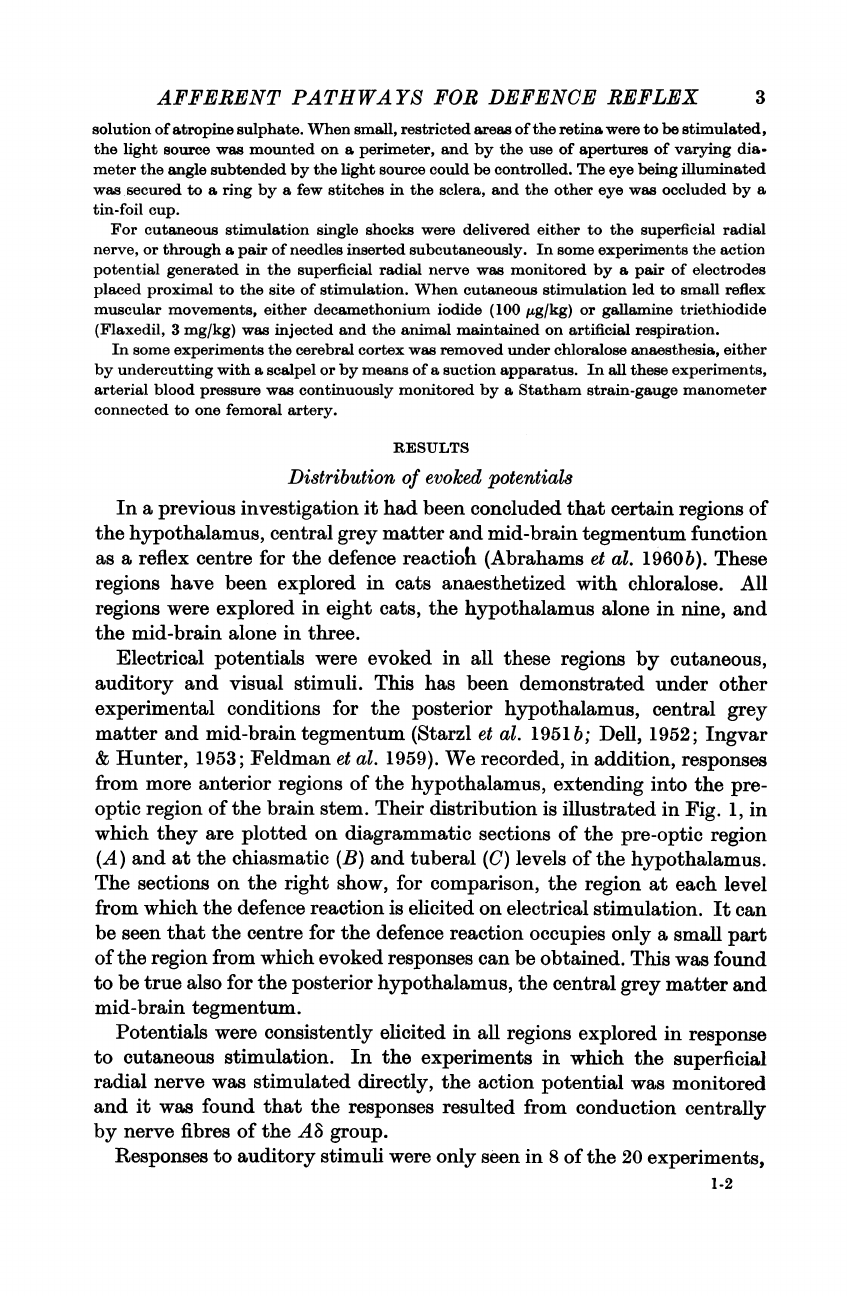
AFFERENT
PATHWAYS
FOR
DEFENCE
REFLEX
solution
of
atropine
sulphate.
When
small,
restricted
areas
of
the
retina
were
to
be
stimulated,
the
light
source
was
mounted
on
a
perimeter,
and
by
the
use
of
apertures
of
varying
dia-
meter
the
angle
subtended
by
the
light
source
could
be
controlled.
The
eye
being
illuminated
was
secured
to
a
ring
by
a
few
stitches
in
the
sclera,
and
the
other
eye
was
occluded
by
a
tin-foil
cup.
For
cutaneous
stimulation
single
shocks
were
delivered
either
to
the
superficial
radial
nerve,
or
through
a
pair
of
needles
inserted
subcutaneously.
In
some
experiments
the
action
potential
generated
in
the
superficial
radial
nerve
was
monitored
by
a
pair
of
electrodes
placed
proximal
to
the
site
of
stimulation.
When
cutaneous
stimulation
led
to
small
reflex
muscular
movements,
either
decamethonium
iodide
(100
,ug/kg)
or
gallamine
triethiodide
(Flaxedil,
3
mg/kg)
was
injected
and
the
animal
maintained
on
artificial
respiration.
In
some
experiments
the
cerebral
cortex
was
removed
under
chloralose
anaesthesia,
either
by
undercutting
with
a
scalpel
or
by
means
of
a
suction
apparatus.
In
all
these
experiments,
arterial
blood
pressure
was
continuously
monitored
by
a
Statham
strain-gauge
manometer
connected
to
one
femoral
artery.
RESULTS
Distribution
of
evoked
potentials
In
a
previous
investigation
it
had
been
concluded
that
certain
regions
of
the
hypothalamus,
central
grey
matter
and
mid-brain
tegmentum
function
as
a
reflex
centre
for
the
defence
reactioh
(Abrahams
et
al.
1960b).
These
regions
have
been
explored
in
cats
anaesthetized
with
chloralose.
All
regions
were
explored
in
eight
cats,
the
hypothalamus
alone
in
nine,
and
the
mid-brain
alone
in
three.
Electrical
potentials
were
evoked
in
all
these
regions
by
cutaneous,
auditory
and
visual
stimuli.
This
has
been
demonstrated
under
other
experimental
conditions
for
the
posterior
hypothalamus,
central
grey
matter
and
mid-brain
tegmentum
(Starzl
et
al.
1951
b;
Dell,
1952;
Ingvar
&
Hunter,
1953;
Feldman
et
al.
1959).
We
recorded,
in
addition,
responses
from
more
anterior
regions
of
the
hypothalamus,
extending
into
the
pre-
optic
region
of
the
brain
stem.
Their
distribution
is
illustrated
in
Fig.
1,
in
which
they
are
plotted
on
diagrammatic
sections
of
the
pre-optic
region
(A)
and
at
the
chiasmatic
(B)
and
tuberal
(C)
levels
of
the
hypothalamus.
The
sections
on
the
right
show,
for
comparison,
the
region
at
each
level
from
which
the
defence
reaction
is
elicited
on
electrical
stimulation.
It
can
be
seen
that
the
centre
for
the
defence
reaction
occupies
only
a
small
part
of
the
region
from
which
evoked
responses
can
be
obtained.
This
was
found
to
be
true
also
for
the
posterior
hypothalamus,
the
central
grey
matter
and
mid-brain
tegmentum.
Potentials
were
consistently
elicited
in
all
regions
explored
in
response
to
cutaneous
stimulation.
In
the
experiments
in
which
the
superficial
radial
nerve
was
stimulated
directly,
the
action
potential
was
monitored
and
it
was
found
that
the
responses
resulted
from
conduction
centrally
by
nerve
fibres
of
the
AS
group.
Responses
to
auditory
stimuli
were
only
seen
in
8
of
the
20
experiments,
1-2
3
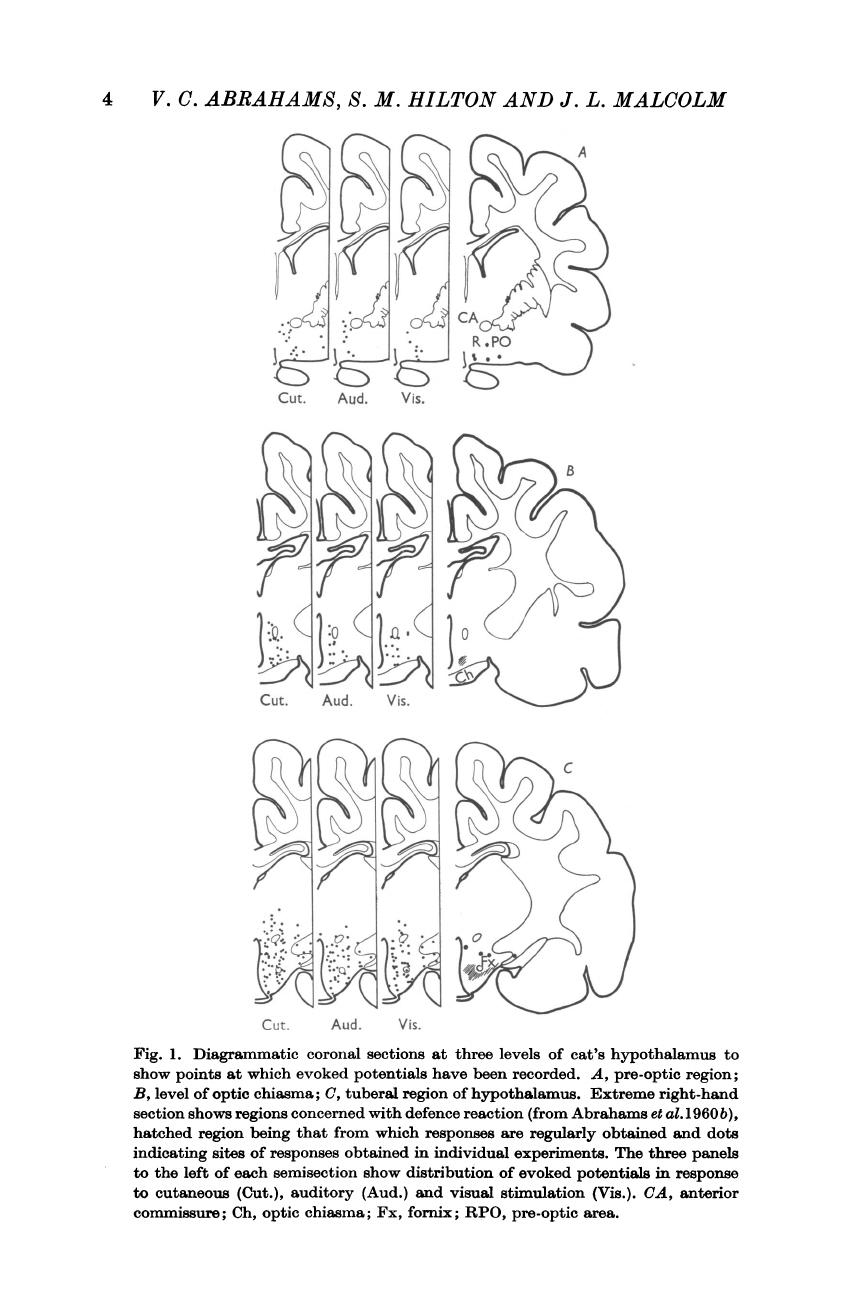
4
V.
C.
ABRAHAMS,
S.
M.
HILTON
AND
J.
L.
MALCOLM
Cut.
Aud.
Vis.
C
Cut.
Aud.
Vis.
Fig.
1.
Diagrammatic
coronal
sections
at
three
levels
of
cat's
hypothalamus
to
show
points
at
which
evoked
potentials
have
been
recorded.
A,
pre-optic
region;
B,
level
of
optic
chiasma;
C,
tuberal
region
of
hypothalamus.
Extreme
right-hand
section
shows
regions
concerned
with
defence
reaction
(from
Abrahams
et
al.
1960b),
hatched
region
being
that
from
which
responses
are
regularly
obtained
and
dots
indicating
sites
of
responses
obtained
in
individual
experiments.
The
three
panels
to
the
left
of
each
semisection
show
distribution
of
evoked
potentials
in
response
to
cutaneous
(Cut.),
auditory
(Aud.)
and
visual
stimulation
(Vis.).
CA,
anterior
commissure;
Ch,
optic
chiasma;
Fx,
fornix;
RPO,
pre-optic
area.
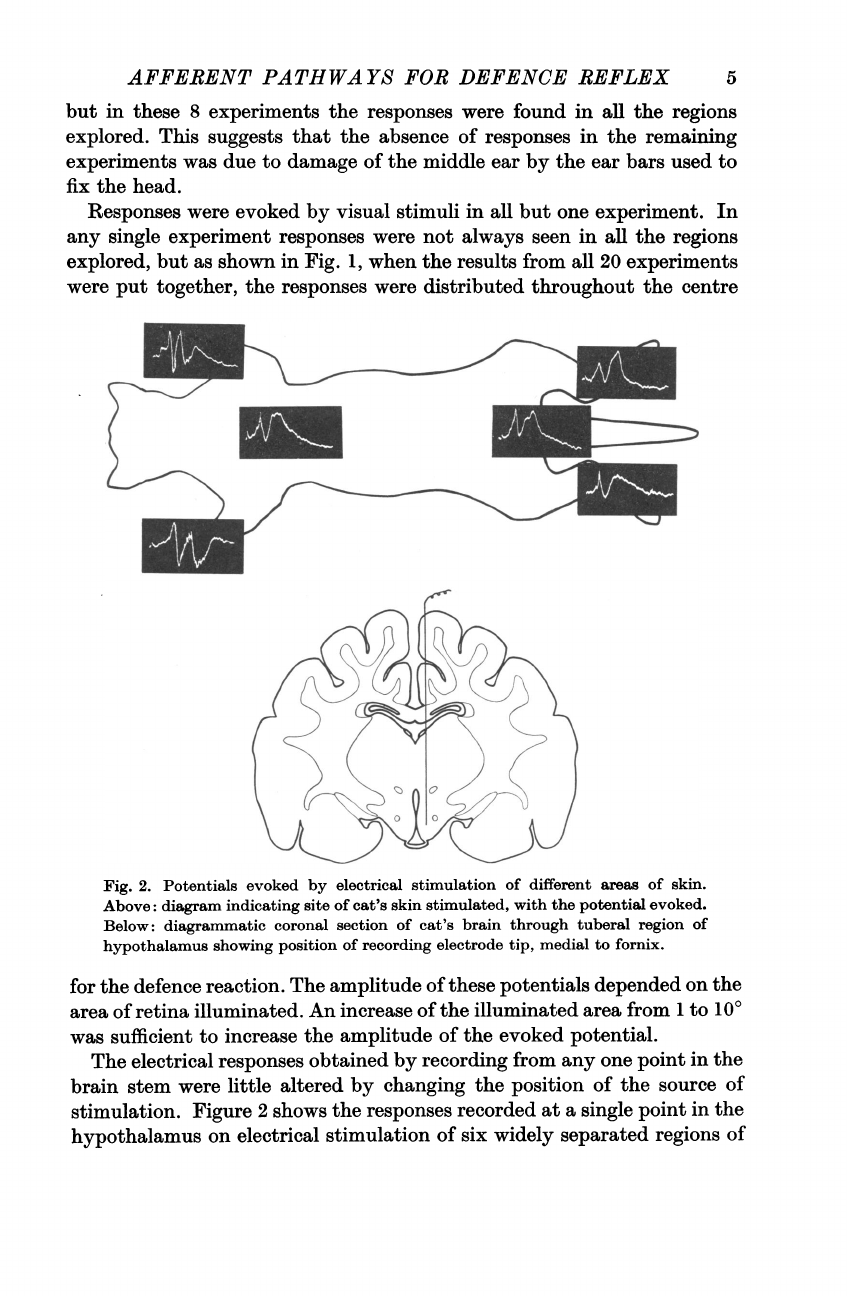
AFFERENT
PATH
WAYS
FOR
DEFENCE
REFLEX
but
in
these
8
experiments
the
responses
were
found
in
all
the
regions
explored.
This
suggests
that
the
absence
of
responses
in
the
remaining
experiments
was
due
to
damage
of
the
middle
ear
by
the
ear
bars
used
to
fix
the
head.
Responses
were
evoked
by
visual
stimuli
in
all
but
one
experiment.
In
any
single
experiment
responses
were
not
always
seen
in
all
the
regions
explored,
but
as
shown
in
Fig.
1,
when
the
results
from
all
20
experiments
were
put
together,
the
responses
were
distributed
throughout
the
centre
.~~~~
Fig.
2.
Potentials
evoked
by
electrical
stimulation
of
different
areas
of
skin.
Above:
diagram
indicating
site
of
cat's
skin
stimulated,
with
the
potential
evoked.
Below:
diagrammatic
coronal
section
of
cat's
brain
through
tuberal
region
of
hypothalamus
showing
position
of
recording
electrode
tip,
medial
to
fornix.
for
the
defence
reaction.
The
amplitude
of
these
potentials
depended
on
the
area
of
retina
illuminated.
An
increase
of
the
illuminated
area
from
1
to
100
was
sufficient
to
increase
the
amplitude
of
the
evoked
potential.
The
electrical
responses
obtained
by
recording
from
any
one
point
in
the
brain
stem
were
little
altered
by
changing
the
position
of
the
source
of
stimulation.
Figure
2
shows
the
responses
recorded
at
a
single
point
in
the
hypothalamus
on
electrical
stimulation
of
six
widely
separated
regions
of
5
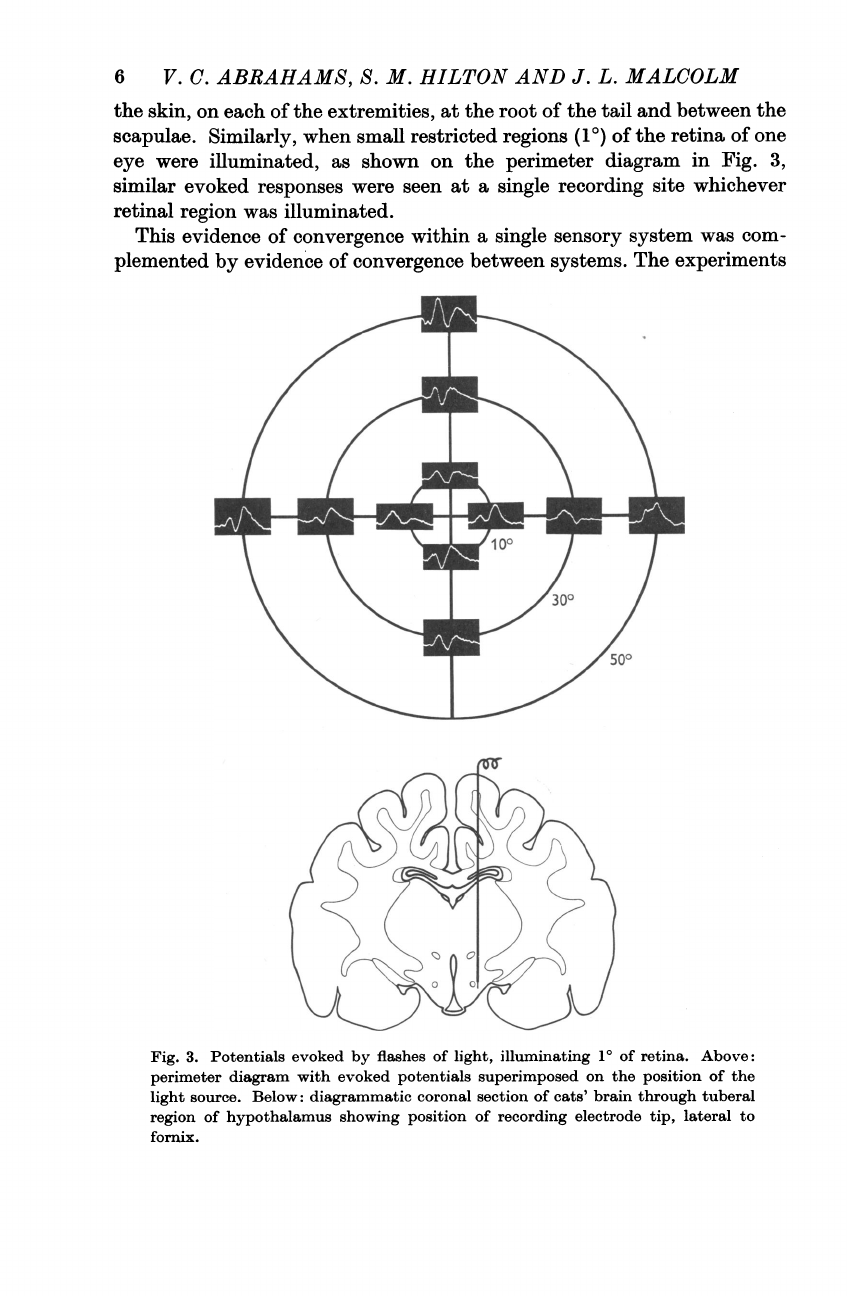
6
V.
C.
ABRAHAMS,
S.
M.
HILTON
AND
J.
L.
MALCOLM
the
skin,
on
each
of
the
extremities,
at
the
root
of
the
tail
and
between
the
scapulae.
Similarly,
when
small
restricted
regions
(10)
of
the
retina
of
one
eye
were
illuminated,
as
shown
on
the
perimeter
diagram
in
Fig.
3,
similar
evoked
responses
were
seen
at
a
single
recording
site
whichever
retinal
region
was
illuminated.
This
evidence
of
convergence
within
a
single
sensory
system
was
com-
plemented
by
evidence
of
convergence
between
systems.
The
experiments
Fig.
3.
Potentials
evoked
by
flashes
of
light,
illuminating
10
of
retina.
Above:
perimeter
diagram
with
evoked
potentials
superimposed
on
the
position
of
the
light
source.
Below:
diagrammatic
coronal
section
of
cats'
brain
through
tuberal
region
of
hypothalamus
showing
position
of
recording
electrode
tip,
lateral
to
fornix.
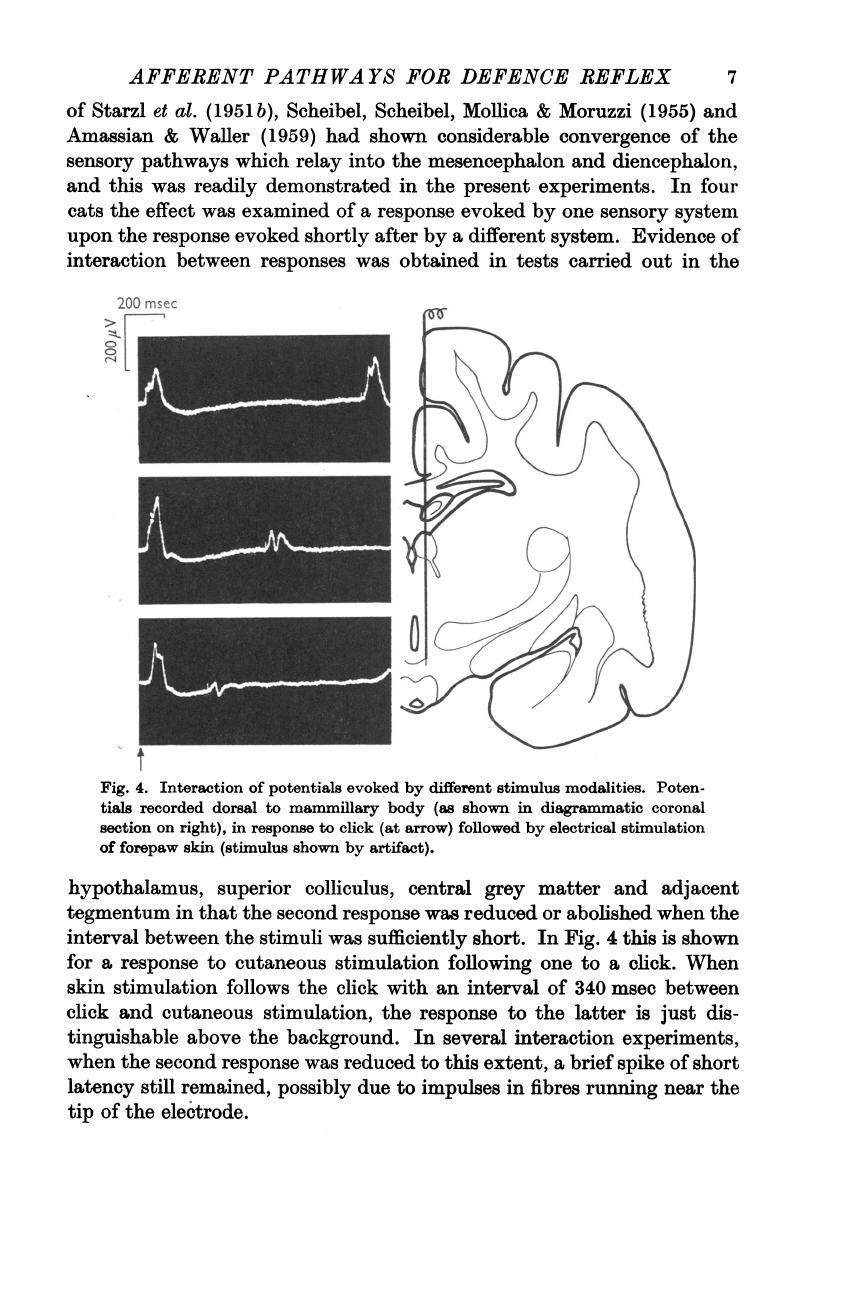
AFFERENT
PATHWAYS
FOR
DEFENCE
REFLEX
of
Starzl
et
al.
(1951
b),
Scheibel,
Scheibel,
Mollica
&
Moruzzi
(1955)
and
Amassian
&
Wailer
(1959)
had
shown
considerable
convergence
of
the
sensory
pathways
which
relay
into
the
mesencephalon
and
diencephalon,
and
this
was
readily
demonstrated
in
the
present
experiments.
In
four
cats
the
effect
was
examined
of
a
response
evoked
by
one
sensory
system
upon
the
response
evoked
shortly
after
by
a
different
system.
Evidence
of
interaction
between
responses
was
obtained
in
tests
carried
out
in
the
200
msec
~~~~~~~"A
Fig.
4.
Interaction
of
potentials
evoked
by
different
stimulus
modalities.
Poten-
tials
recorded
dorsal
to
mammillary
body
(as
shown
in
diagrammatic
coronal
section
on
right),
in
response
to
click
(at
arrow)
followed
by
electrical
stimulation
of
forepaw
skin
(stimulus
shown
by
artifact).
hypothalamus,
superior
colliculus,
central
grey
matter
and
adjacent
tegmentum
in
that
the
second
response
was
reduced
or
abolished
when
the
interval
between
the
stimuli
was
sufficiently
short.
In
Fig.
4
this
is
shown
for
a
response
to
cutaneous
stimulation
following
one
to
a
click.
When
skin
stimulation
follows
the
click
with
an
interval
of
340
msec
between
click
and
cutaneous
stimulation,
the
response
to
the
latter
is
just
dis-
tinguishable
above
the
background.
In
several
interaction
experiments,
when
the
second
response
was
reduced
to
this
extent,
a
brief
spike
of
short
latency
still
remained,
possibly
due
to
impulses
in
fibres
running
near
the
tip
of
the
electrode.
7
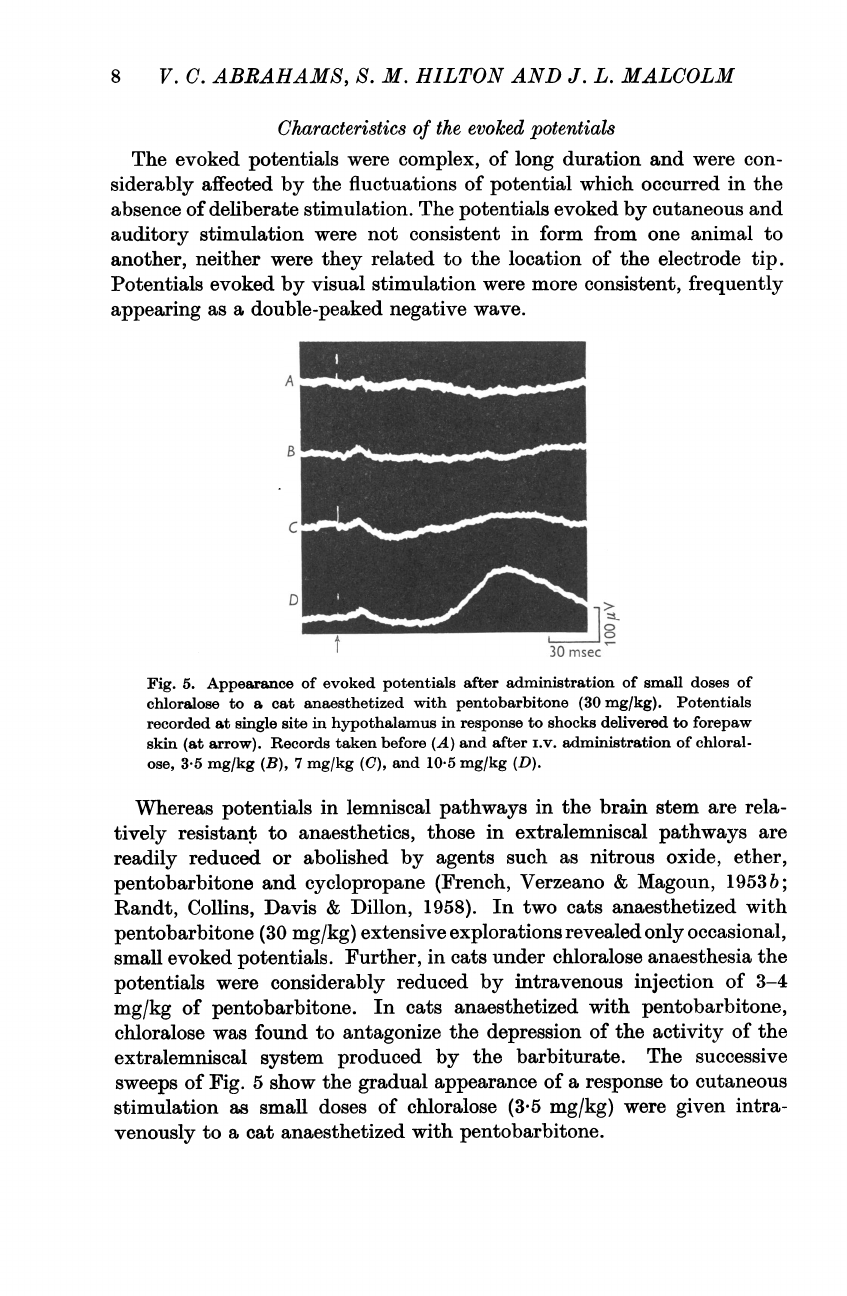
8
V.
C.
ABBAHAMS,
S.
M.
HILTON
AND
J.
L.
MALCOLM
Characteristics
of
the
evoked
potentials
The
evoked
potentials
were
complex,
of
long
duration
and
were
con-
siderably
affected
by
the
fluctuations
of
potential
which
occurred
in
the
absence
of
deliberate
stimulation.
The
potentials
evoked
by
cutaneous
and
auditory
stimulation
were
not
consistent
in
form
from
one
animal
to
another,
neither
were
they
related
to
the
location
of
the
electrode
tip.
Potentials
evoked
by
visual
stimulation
were
more
consistent,
frequently
appearing
as
a
double-peaked
negative
wave.
A
_
B
C
D_|
A
C)1°
30
nisec
Fig.
5.
Appearance
of
evoked
potentials
after
administration
of
small
doses of
chloralose
to
a
cat
anaesthetized
with
pentobarbitone
(30
mg/kg).
Potentials
recorded
at
single
site
in
hypothalamus
in
response
to
shocks
delivered
to
forepaw
skin
(at
arrow).
Records
taken
before
(A)
and
after
i.v.
administration
of
chloral-
ose,
3-5
mg/kg
(B),
7
mg/kg
(C),
and
10-5
mg/kg
(D).
Whereas
potentials
in
lemniscal
pathways
in
the
brain
stem
are
rela-
tively
resistant
to
anaesthetics,
those
in
extralemniscal
pathways
are
readily
reduced
or
abolished
by
agents
such
as
nitrous
oxide,
ether,
pentobarbitone
and
cyclopropane
(French,
Verzeano
&
Magoun,
1953
b;
Randt,
Collins,
Davis
&
Dillon,
1958).
In
two
cats
anaesthetized
with
pentobarbitone
(30
mg/kg)
extensive
explorations
revealed
only
occasional,
small
evoked
potentials.
Further,
in
cats
under
chloralose
anaesthesia
the
potentials
were
considerably
reduced
by
intravenous
injection
of
3-4
mg/kg
of
pentobarbitone.
In
cats
anaesthetized
with
pentobarbitone,
chloralose
was
found
to
antagonize
the
depression
of
the
activity
of
the
extralemniscal
system
produced
by
the
barbiturate.
The
successive
sweeps
of
Fig.
5
show
the
gradual
appearance
of
a
response
to
cutaneous
stimulation
as
small
doses
of
chloralose
(3-5
mg/kg)
were
given
intra-
venously
to
a
cat
anaesthetized
with
pentobarbitone.

AFFERENT
PATH
WAYS
FOR
DEFENCE
REFLEX
This
is
evidence
of
a
facilitatory
effect
of
chloralose
on
the
neurones
of
the
afferent
pathway,
but,
in
addition,
these
experiments
show
one
of
the
sites
where
chloralose
exerts
a
blocking
action.
Electrical
stimulation
via
electrodes
stereotactically
placed
in
the
same
brain-stem
regions
readily
elicits
all
the
autonomic
components
of
the
defence
reaction
in
cats
under
chloralose.
Nevertheless,
even
following
intense
cutaneous
stimulation
in
such
cats
it
is
not
possible
to
obtain
the
autonomic
components
of
the
defence
reaction
reflexly
(Abrahams
et
al.
1960
b).
It
therefore
appears
that
one
of
the
sites
of
action
of
chloralose
is
at
the
synapses
between
afferent
and
efferent
pathways
in
the
brain-stem
centre
for
the
defence
reaction.
Latencies
of
evoked
potentials
In
individual
animals
differences
were
recorded
in
the
latencies
of
the
responses,
according
to
the
site
of
the
recording
electrode
and
the
sensory
system
being
activated.
The
latencies
also
depended
on
the
polarity
of
the
initial
component,
being
shorter
when
this
was
negative-going.
In
19
experiments,
in
which
latencies
were
measured
of
responses
to
electrical
stimulation
of
the
skin
of
the
fore
or
hind
limbs,
the
values
lay
between
6
and
16*5
msec.
In
most
experiments
the
latencies
tended
to
be
shorter
in
the
central
grey
matter
than
in
the
hypothalamus,
and
so
in
two
cats
a
large
number
of
observations
were
made
of
latencies
in
both
brain-stem
regions.
Only
responses
with
an
initial
negative-going
component
were
compared.
The
mean
value
for
the
latency
in
the
central
grey
matter
was
shorter
than
that
in
the
hypothalamus
by
3-9
msec
(t
=
8-696,
P
<
0-001)
in
one
experiment
and
6*1
msec
(t
=
11*05,
P
<
0.001)
in
the
other.
The
latencies
of
the
responses
evoked
in
the
hypothalamus
were,
of
course,
longer
than
those
recorded
in
the
somatic
sensory
cortex.
In
2
experiments
potentials
were
recorded
simultaneously
at
a
point
in
the
hypothalamus
and
at
a
site
on
the
precruciate
region
of
the
cerebral
cortex
where
the
shortest
latencies
were
found.
The
latency
of
the
cortical
responses
was
always
less
than
that
of
the
corresponding
hypothalamic
response,
the
mean
difference
being
4
msec
in
one
experiment
and
5
1
msec
in
the
other.
The
latencies
of
the
responses
to
auditory
stimulation
ranged
from
10
to
22
msec,
there
being
no
consistent
difference
in
the
values
recorded
in
the
various
brain-stem
regions.
The
responses
to
visual
stimulation
appeared
with
longer
latencies-from
40
to
50
msec
in
all
regions-except
for
the
hypothalamus
where
they
were
as
short
as
30
msec
in
one
experiment
and
as
long
as
68
msec
in
another.
Evoked
potentials
after
removal
of
the
cerebral
cortex
and
basal
ganglia
In
four
cats
under
chloralose
anaesthesia
decerebration
was
performed
at
a
high
level
with
the
intention
of
removing
most
of
the
brain
lying
9
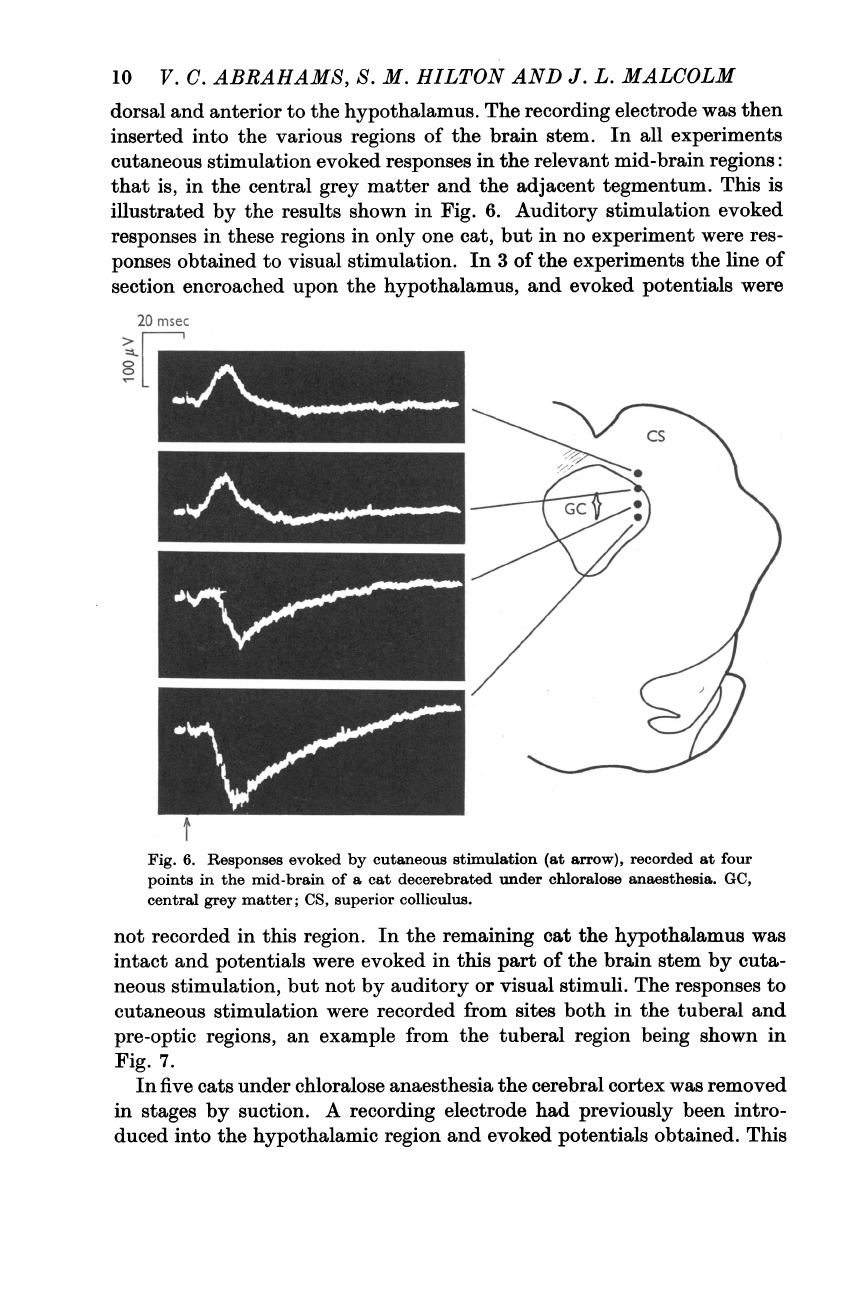
10
V.
C.
ABRAHAMS,
S.
M.
HILTON
AND
J.
L.
MALCOLM
dorsal
and
anterior
to
the
hypothalamus.
The
recording
electrode
was
then
inserted
into
the
various
regions
of
the
brain
stem.
In
all
experiments
cutaneous
stimulation
evoked
responses
in
the
relevant
mid-brain
regions:
that
is,
in
the
central
grey
matter
and
the
adjacent
tegmentum.
This
is
illustrated
by
the
results
shown
in
Fig.
6.
Auditory
stimulation
evoked
responses
in
these
regions
in
only
one
cat,
but
in
no
experiment
were
res-
ponses
obtained
to
visual
stimulation.
In
3
of
the
experiments
the
line
of
section
encroached
upon
the
hypothalamus,
and
evoked
potentials
were
20
msec
8
T
Fig.
6.
Responses
evoked
by
cutaneous
stimulation
(at
arrow),
recorded
at
four
points
in
the
mid-brain
of
a
cat
decerebrated
under
chloralose
anaesthesia.
GC,
central
grey
matter;
CS,
superior
colliculus.
not
recorded
in
this
region.
In
the
remaining
cat
the
hypothalamus
was
intact
and
potentials
were
evoked
in
this
part
of
the
brain
stem
by
cuta-
neous
stimulation,
but
not
by
auditory
or
visual
stimuli.
The
responses
to
cutaneous
stimulation
were
recorded
from
sites
both
in
the
tuberal
and
pre-optic
regions,
an
example
from
the
tuberal
region
being
shown
in
Fig.
7.
In
five
cats
under
chloralose
anaesthesia
the
cerebral
cortex
was
removed
in
stages
by
suction.
A
recording
electrode
had
previously
been
intro-
duced
into
the
hypothalamic
region
and
evoked
potentials
obtained.
This
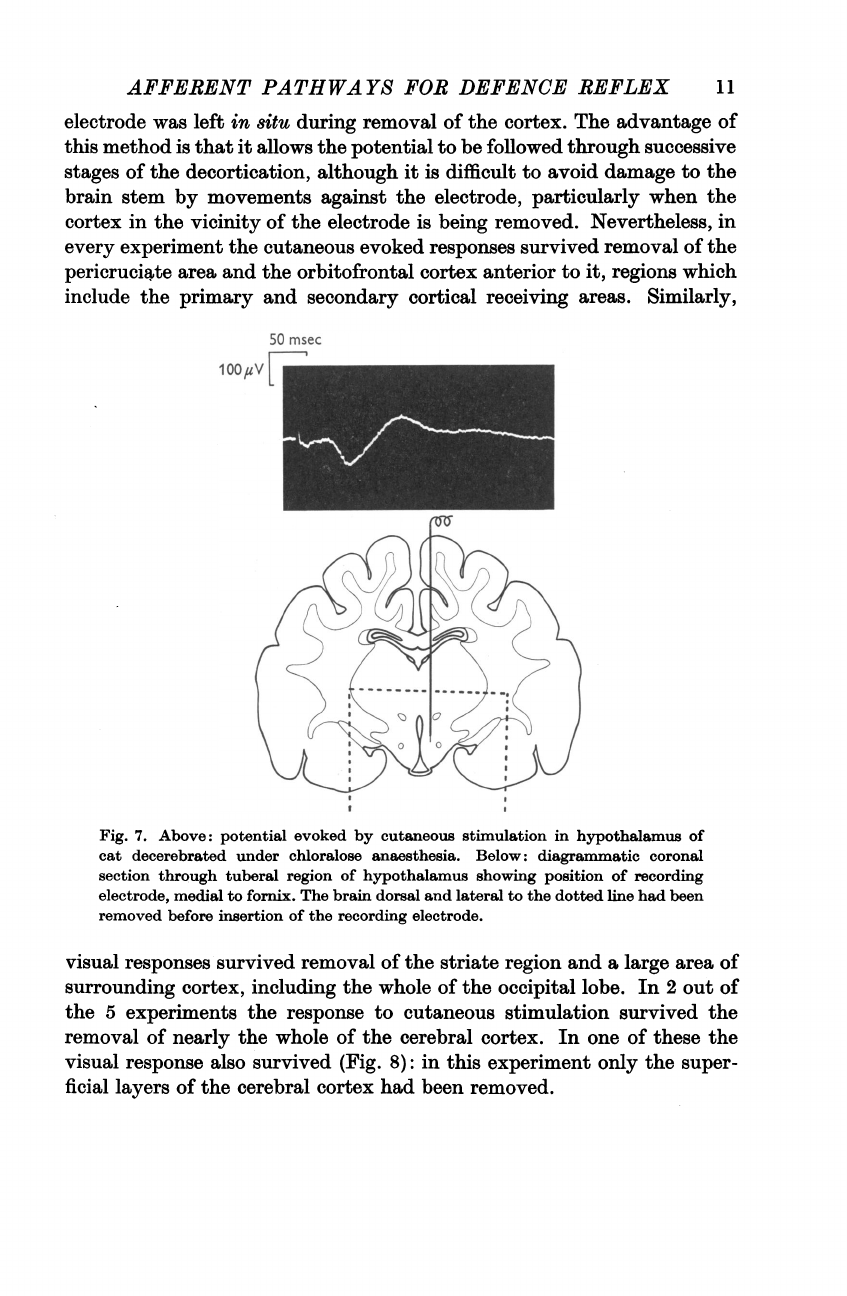
AFFERENT
PATHWAYS
FOR
DEFENCE
REFLEX
electrode
was
left
in
situ
during
removal
of
the
cortex.
The
advantage
of
this
method
is
that
it
allows
the
potential
to
be
followed
through
successive
stages
of
the
decortication,
although
it
is
difficult
to
avoid
damage
to
the
brain
stem
by
movements
against
the
electrode,
particularly
when
the
cortex
in
the
vicinity
of
the
electrode
is
being
removed.
Nevertheless,
in
every
experiment
the
cutaneous
evoked
responses
survived
removal
of
the
pericruciate
area
and
the
orbitofrontal
cortex
anterior
to
it,
regions
which
include
the
primary
and
secondary
cortical
receiving
areas.
Similarly,
50
msec
100#V
Fig.
7.
Above:
potential
evoked
by
cutaneous
stimulation
in
hypothalamus
of
cat
decerebrated
under
chloralose
anaesthesia.
Below:
diagrammatic
coronal
section
through
tuberal
region
of
hypothalamus
showing
position
of
recording
electrode,
medial
to
fornix.
The
brain
dorsal
and
lateral
to
the
dotted
line
had
been
removed
before
insertion
of
the
recording
electrode.
visual
responses
survived
removal
of
the
striate
region
and
a
large
area
of
surrounding
cortex,
including
the
whole
of
the
occipital
lobe.
In
2
out
of
the
5
experiments
the
response
to
cutaneous
stimulation
survived
the
removal
of
nearly
the
whole
of
the
cerebral
cortex.
In
one
of
these
the
visual
response
also
survived
(Fig.
8):
in
this
experiment
only
the
super-
ficial
layers
of
the
cerebral
cortex
had
been
removed.
11
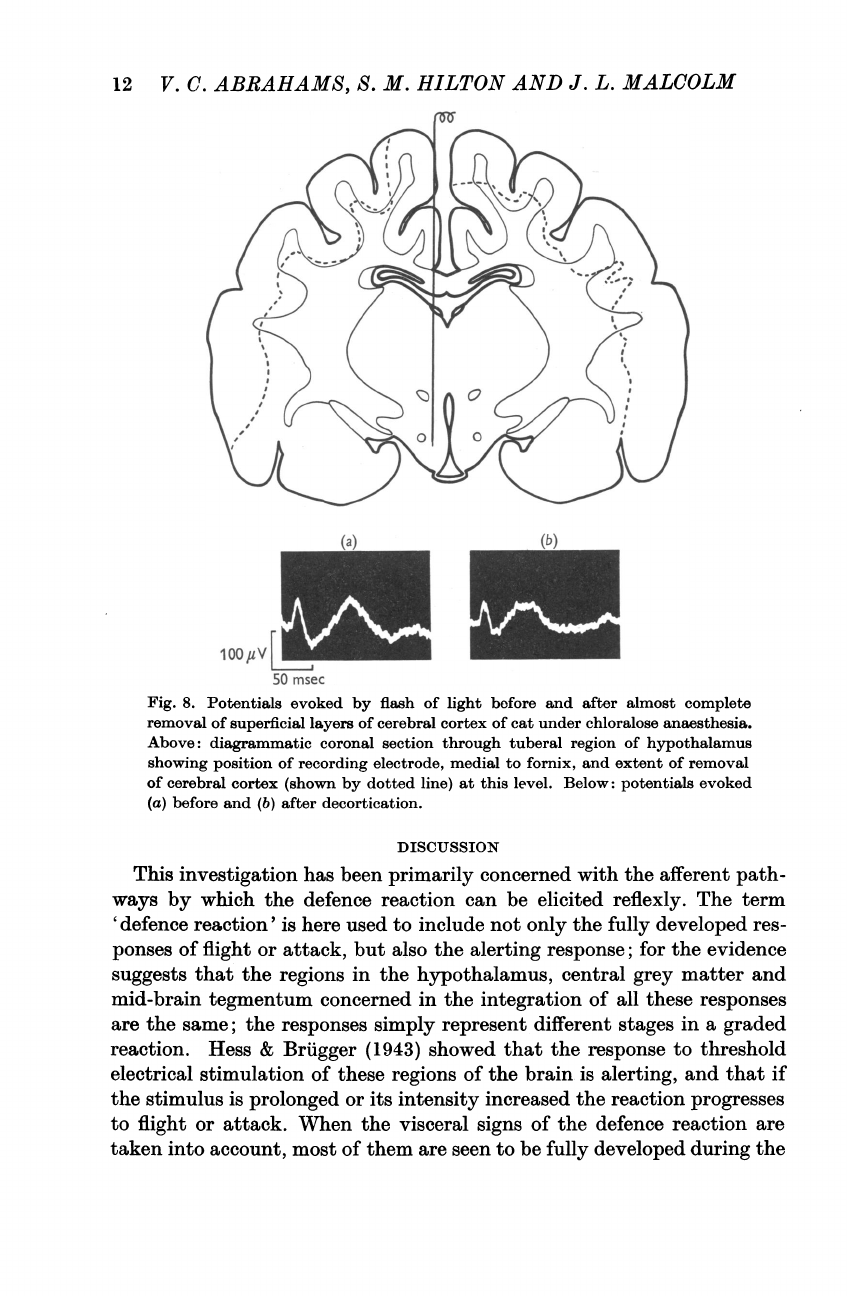
12
V.
C.
ABRAHAMS,
S.
M.
HILTON
AND
J.
L.
MALCOLM
50
msec
Fig.
8.
Potentials
evoked
by
flash
of
light
before
and
after
almost
complete
removal
of
superficial
layers
of
cerebral
cortex
of
cat
under
chloralose
anaesthesia.
Above:
diagrammatic
coronal
section
through
tuberal
region
of
hypothalamus
showing
position
of
recording
electrode,
medial
to
fornix,
and
extent
of
removal
of
cerebral
cortex
(shown
by
dotted
line)
at
this
level.
Below:
potentials
evoked
(a)
before
and
(b)
after
decortication.
DISCUSSION
This
investigation
has
been
primarily
concerned
with
the
afferent
path-
ways
by
which
the
defence
reaction
can
be
elicited
reflexly.
The
term
'defence
reaction'
is
here
used
to
include
not
only
the
fully
developed
res-
ponses
of
flight
or
attack,
but
also
the
alerting
response;
for
the
evidence
suggests
that
the
regions
in
the
hypothalamus,
central
grey
matter
and
mid-brain
tegmentum
concerned
in
the
integration
of
all
these
responses
are
the
same;
the
responses
simply
represent
different
stages
in
a
graded
reaction.
Hess
&
Briigger
(1943)
showed
that
the
response
to
threshold
electrical
stimulation
of
these
regions
of
the
brain
is
alerting,
and
that
if
the
stimulus
is
prolonged
or
its
intensity
increased
the
reaction
progresses
to
flight
or
attack.
When
the
visceral
signs
of
the
defence
reaction
are
taken
into
account,
most
of
them
are
seen
to
be
fully
developed
during
the
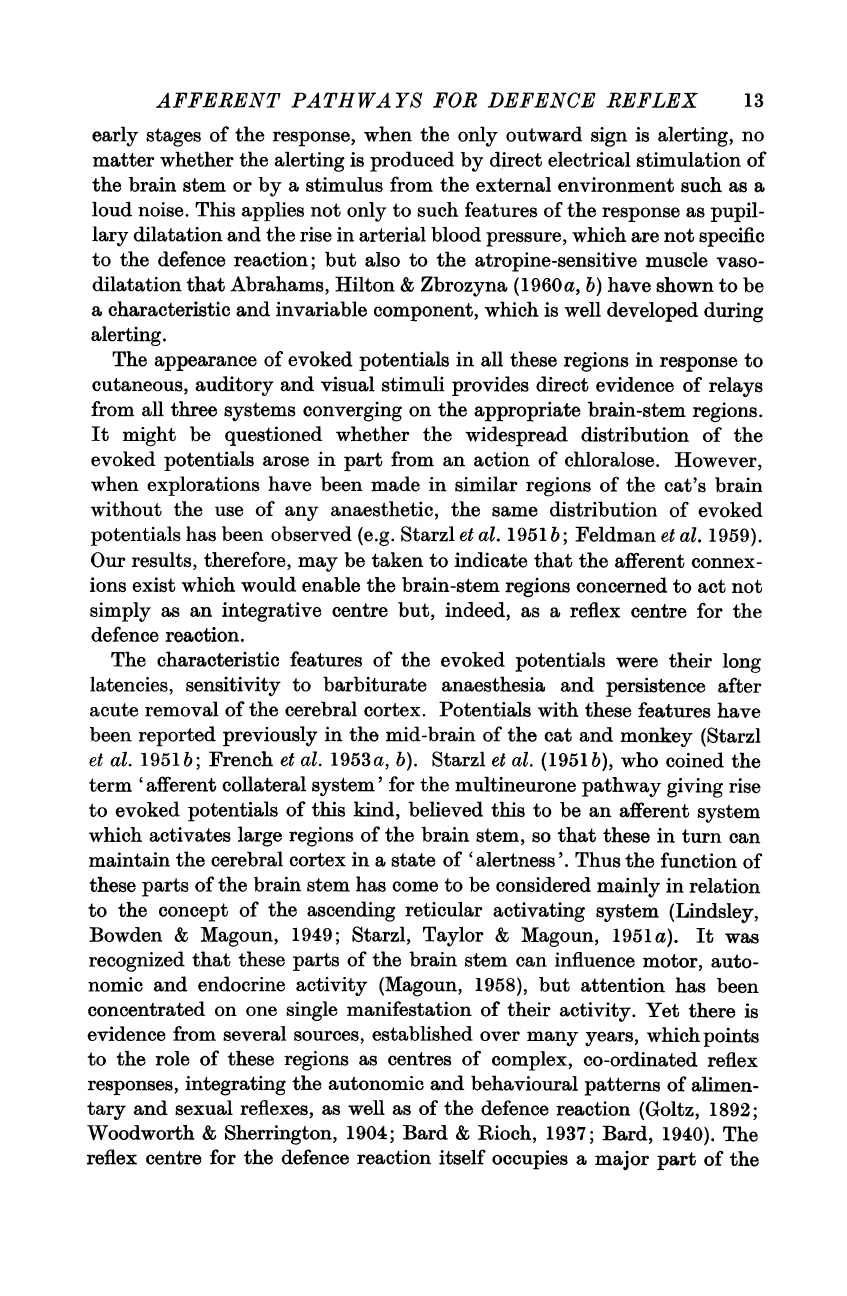
AFFERENT
PATHWAYS
FOR
DEFENCE
REFLEX
early
stages
of
the
response,
when
the
only
outward
sign
is
alerting,
no
matter
whether
the
alerting
is
produced
by
direct
electrical
stimulation
of
the
brain
stem
or
by
a
stimulus
from
the
external
environment
such
as
a
loud
noise.
This
applies
not
only
to
such
features
of
the
response
as
pupil-
lary
dilatation
and
the
rise
in
arterial
blood
pressure,
which
are
not
specific
to
the
defence
reaction;
but
also
to
the
atropine-sensitive
muscle
vaso-
dilatation
that
Abrahams,
Hilton
&
Zbrozyna
(1960
a,
b)
have
shown
to
be
a
characteristic
and
invariable
component,
which
is
well
developed
during
alerting.
The
appearance
of
evoked
potentials
in
all
these
regions
in
response
to
cutaneous,
auditory
and
visual
stimuli
provides
direct
evidence
of
relays
from
all
three
systems
converging
on
the
appropriate
brain-stem
regions.
It
might
be
questioned
whether
the
widespread
distribution
of
the
evoked
potentials
arose
in
part
from
an
action
of
chloralose.
However,
when
explorations
have
been
made
in
similar
regions
of
the
cat's
brain
without
the
use
of
any
anaesthetic,
the
same
distribution
of
evoked
potentials
has
been
observed
(e.g.
Starzl
et
al.
1951
b;
Feldman
et
at.
1959).
Our
results,
therefore,
may
be
taken
to
indicate
that
the
afferent
connex-
ions
exist
which
would
enable
the
brain-stem
regions
concerned
to
act
not
simply
as
an
integrative
centre
but,
indeed,
as
a
reflex
centre
for
the
defence
reaction.
The
characteristic
features
of
the
evoked
potentials
were
their
long
latencies,
sensitivity
to
barbiturate
anaesthesia
and
persistence
after
acute
removal
of
the
cerebral
cortex.
Potentials
with
these
features
have
been
reported
previously
in
the
mid-brain
of
the
cat
and
monkey
(Starzl
et
al.
1951b;
French
et
at.
1953a,
b).
Starzl
et
al.
(1951b),
who
coined
the
term
'afferent
collateral
system'
for
the
multineurone
pathway
giving
rise
to
evoked
potentials
of
this
kind,
believed
this
to
be
an
afferent
system
which
activates
large
regions
of
the
brain
stem,
so
that
these
in
turn
can
maintain
the
cerebral
cortex
in
a
state
of
'alertness'.
Thus
the
function
of
these
parts
of
the
brain
stem
has
come
to
be
considered
mainly
in
relation
to
the
concept
of the
ascending
reticular
activating
system
(Lindsley,
Bowden
&
Magoun,
1949;
Starzl,
Taylor
&
Magoun,
1951
a).
It
was
recognized
that
these
parts
of
the
brain
stem
can
influence
motor,
auto-
nomic
and
endocrine
activity
(Magoun,
1958),
but
attention
has
been
concentrated
on
one
single
manifestation
of
their
activity.
Yet
there
is
evidence
from
several
sources,
established
over
many
years,
which
points
to
the
role
of
these
regions
as
centres
of
complex,
co-ordinated
reflex
responses,
integrating
the
autonomic
and
behavioural
patterns
of
alimen-
tary
and
sexual
reflexes,
as
well
as
of
the
defence
reaction
(Goltz,
1892;
Woodworth
&
Sherrington,
1904;
Bard
&
Rioch,
1937;
Bard,
1940).
The
reflex
centre
for
the
defence
reaction
itself
occupies
a
major
part
of
the
13
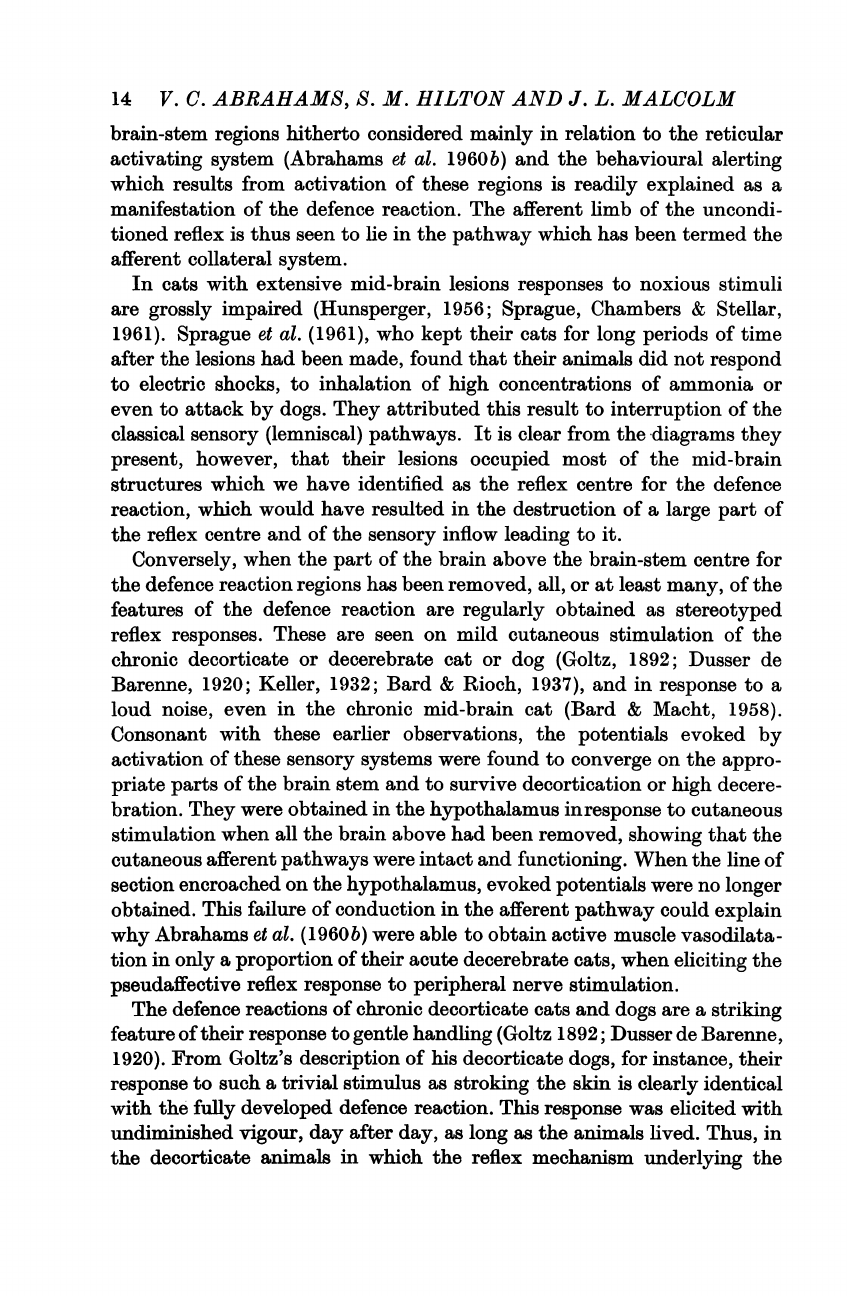
14
V.
C.
ABRAHAMS,
S.
M.
HILTON
AND
J.
L.
MALCOLM
brain-stem
regions
hitherto
considered
mainly
in
relation
to
the
reticular
activating
system
(Abrahams
et
al.
1960b)
and
the
behavioural
alerting
which
results
from
activation
of
these
regions
is
readily
explained
as
a
manifestation
of
the
defence
reaction.
The
afferent
limb
of
the
uncondi-
tioned
reflex
is
thus
seen
to
lie
in
the
pathway
which
has
been
termed
the
afferent
collateral
system.
In
cats
with
extensive
mid-brain
lesions
responses
to
noxious
stimuli
are
grossly
impaired
(Hunsperger,
1956;
Sprague,
Chambers
&
Stellar,
1961).
Sprague
et
al.
(1961),
who
kept
their
cats
for
long
periods of
time
after
the
lesions
had
been
made,
found
that
their
animals
did
not
respond
to
electric
shocks,
to
inhalation
of
high
concentrations
of
ammonia
or
even
to
attack
by
dogs.
They
attributed
this
result
to
interruption
of
the
classical
sensory
(lemniscal)
pathways.
It
is
clear
from
the
diagrams
they
present,
however,
that
their
lesions
occupied
most
of
the
mid-brain
structures
which
we
have
identified
as
the
reflex
centre
for
the
defence
reaction,
which
would
have
resulted
in
the
destruction
of
a
large
part
of
the
reflex
centre
and
of
the
sensory
inflow
leading
to
it.
Conversely,
when
the
part
of
the
brain
above
the
brain-stem
centre
for
the
defence
reaction
regions
has
been
removed,
all,
or
at
least
many,
of
the
features
of
the
defence
reaction
are
regularly
obtained
as
stereotyped
reflex
responses.
These
are
seen
on
mild
cutaneous
stimulation
of
the
chronic
decorticate
or
decerebrate
cat
or
dog
(Goltz,
1892;
Dusser
de
Barenne,
1920;
Keller,
1932;
Bard
&
Rioch,
1937),
and
in
response
to
a
loud
noise,
even
in
the
chronic
mid-brain
cat
(Bard
&
Macht,
1958).
Consonant
with
these
earlier
observations,
the
potentials
evoked
by
activation
of
these
sensory
systems
were
found
to
converge
on
the
appro-
priate
parts
of
the
brain
stem
and
to
survive
decortication
or
high
decere-
bration.
They
were
obtained
in
the
hypothalamus
inresponse
to
cutaneous
stimulation
when
all
the
brain
above
had
been
removed,
showing
that
the
cutaneous
afferent
pathways
were
intact
and
functioning.
When
the
line
of
section
encroached
on
the
hypothalamus,
evoked
potentials
were
no
longer
obtained.
This
failure
of
conduction
in
the
afferent
pathway
could
explain
why
Abrahams
et
al.
(1960b)
were
able
to
obtain
active
muscle
vasodilata-
tion
in
only
a
proportion
of
their
acute
decerebrate
cats,
when
eliciting
the
pseudaffective
reflex
response
to
peripheral
nerve
stimulation.
The
defence
reactions
of
chronic
decorticate
cats
and
dogs
are
a
striking
feature
of
their
response
to
gentle
handling
(Goltz
1892;
Dusser
de
Barenne,
1920).
From
Goltz's
description
of
his
decorticate
dogs,
for
instance,
their
response
to
such
a
trivial
stimulus
as
stroking
the
skin
is
clearly
identical
with
the
fully
developed
defence
reaction.
This
response
was
elicited
with
undiminished
vigour,
day
after
day,
as
long
as
the
animals
lived.
Thus,
in
the
decorticate
animals
in
which
the
reflex
mechanism
underlying
the
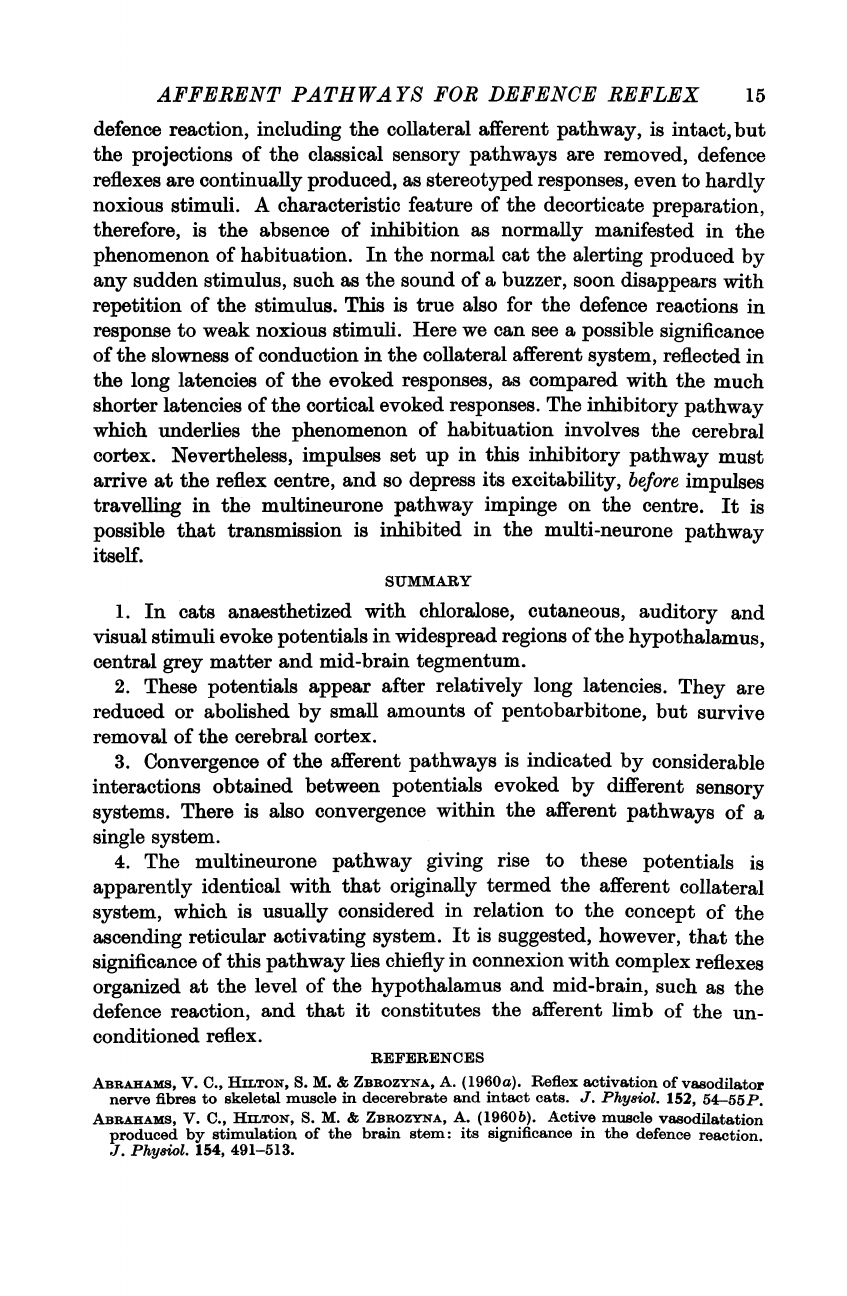
AFFERENT
PATHWAYS
FOR
DEFENCE
REFLEX
defence
reaction,
including
the
collateral
afferent
pathway,
is
intact,
but
the
projections
of
the
classical
sensory
pathways
are
removed,
defence
reflexes
are
continually
produced,
as
stereotyped
responses,
even
to
hardly
noxious
stimuli.
A
characteristic
feature
of
the
decorticate
preparation,
therefore,
is
the
absence
of
inhibition
as
normally
manifested
in
the
phenomenon
of
habituation.
In
the
normal
cat
the
alerting
produced
by
any
sudden
stimulus,
such
as
the
sound
of
a
buzzer,
soon
disappears
with
repetition
of
the
stimulus.
This
is
true
also
for
the
defence
reactions
in
response
to
weak
noxious
stimuli.
Here
we
can
see
a
possible
significance
of
the
slowness
of
conduction
in
the
collateral
afferent
system,
reflected
in
the
long
latencies
of
the
evoked
responses,
as
compared
with
the
much
shorter
latencies
of
the
cortical
evoked
responses.
The
inhibitory
pathway
which
underlies
the
phenomenon
of
habituation
involves
the
cerebral
cortex.
Nevertheless,
impulses
set
up
in
this
inhibitory
pathway
must
arrive
at
the
reflex
centre,
and
so
depress
its
excitability,
before
impulses
travelling
in
the
multineurone
pathway
impinge
on
the
centre.
It
is
possible
that
transmission
is
inhibited
in
the
multi-neurone
pathway
itself.
SUMMARY
1.
In
cats
anaesthetized
with
chloralose,
cutaneous,
auditory
and
visual
stimuli
evoke
potentials
in
widespread
regions
of
the
hypothalamus,
central
grey
matter
and
mid-brain
tegmentum.
2.
These
potentials
appear
after
relatively
long
latencies.
They
are
reduced
or
abolished
by
small
amounts
of
pentobarbitone,
but
survive
removal
of
the
cerebral
cortex.
3.
Convergence
of
the
afferent
pathways
is
indicated
by
considerable
interactions
obtained
between
potentials
evoked
by
different
sensory
systems.
There
is
also
convergence
within
the
afferent
pathways
of
a
single
system.
4.
The
multineurone
pathway
giving
rise
to
these
potentials
is
apparently
identical
with
that
originally
termed
the
afferent
collateral
system,
which
is
usually
considered
in
relation
to
the
concept
of
the
ascending
reticular
activating
system.
It
is
suggested,
however,
that
the
significance
of
this
pathway
lies
chiefly
in
connexion
with
complex
reflexes
organized
at
the
level
of
the
hypothalamus
and
mid-brain,
such
as
the
defence
reaction,
and
that
it
constitutes
the
afferent
limb
of
the
un-
conditioned
reflex.
REFERENCES
ABRAHAMS,
V.
C.,
HiLTON,
S.
M.
&
ZBROZYNA,
A.
(1960a).
Reflex
activation
of
vasodilator
nerve
fibres
to
skeletal
muscle
in
decerebrate
and
intact
cats.
J.
Phy8iol.
152,
54-5p.
ABRAHAMS,
V.
C.,
HITON,
S.
M.
&
ZBROZYNA,
A.
(1960b).
Active
muscle
vasodilatation
produced
by
stimulation
of
the
brain
stem:
its
significance
in
the
defence
reaction.
J.
Phy8iol.
154,
491-513.
15

16
V.
C.
ABRAHAMS,
S.
M.
HILTON
AND
J.
L.
MALCOLM
AMIASSAN,
V.
E.
&
WALLER,
H.
J.
(1959).
Spatiotemporal
patterns
of
activity
in
individual
reticular
neurons.
In
Reticular
Formation
of
the
Brain,
pp.
69-108.
London:
Churchill.
BARD,
P.
(1928).
A
diencephalic
mechanism
for
the
expression
of
rage
with
special
reference
to
the
sympathetic
nervous
sytem.
Amer.
J.
Phyaiol.
84,
490-515.
BARD,
P.
(1940).
The
hypothalamus
and
sexual
behaviour.
Res.
Publ.
nerv.
ment.
Di8.
20,
551-579.
BARD,
P.
&
MACHT,
M.
B.
(1958).
The
behaviour
of
chronically
decerebrate
cats.
In
Neurological
Basis
of
Behaviour,
pp.
57-71.
London:
Churchill.
BARD,
P.
&
RIocH,
D.
McK.
(1937).
A
study
of
four
cats
deprived
of
neocortex
and
addi-
tional
portions
of
the
forebrain.
Johns
Hopk.
Hosp.
Bull.
60,
65-125.
CANNON,
W.
B.
&
BRITTON,
S.
W.
(1925).
Studies
on
the
conditions
of
activity
in
endocrine
glands;
pseudoaffective
medulliadrenal
secretion.
Amer.
J.
Physiol.
72,
283-294.
DELL,
P.
(1952).
Correlations
entre
le
systeme
vegetatif
et
le
systeme
de
la
vie
de
relation.
Mesencephale,
dienc6phale
et
cortex
cerebrale
(1).
J.
Physiol.
Path.
gen.
44,
471-557.
DusSER
DE
BARENNE,
J.
G.
(1920).
Recherches
experimentales
sur
les
fonctions
du
syst6me
nerveux
central,
faites
en
particulier
sur
deux
chats
dont
neopallium
avait
et6
enlev6.
Arch.
neerl.
Physiol.
4,
31-123.
FELDMAN,
S.,
VAN
DER
HEIDE,
C.
S.
&
PORTER,
R.
W.
(1959).
Evoked
potentials
in
the
hypothalamus.
Amer.
J.
Physiol.
196,
1163-1167.
FRENCH,
J.
D.,
VERZEANO,
M.
&
MAGOUN,
H.
W.
(1953a).
An
extralemniscal
sensory
system
in
the
brain.
Arch.
Neurol.
Chicago,
69,
505-518.
FRENCH,
J.
D.,
VERZEANO,
M.
&
MAGOUN,
H.
W.
(1953b).
A
neural
basis
for
the
anaesthetic
state.
Arch.
Neurol.
Chicago,
69,
519-529.
GOLTZ,
F.
M.
(1892).
Der
Hund
ohne
Grosshirn.
Pflug.
Arch.
ges.
Physiol.
51,
570-614.
GREEN,
J.
D.
(1958).
A
simple
micro-electrode
for
recording
from
the
central
nervous
system.
Nature,
Lond.,
182,
962.
HESS,
W.
R.
&
BRUGGER,
M.
(1943).
Das
subcorticale
Zentrum
der
affectiven
Abwehr-
reaktion.
Helv.
physiol.
acta,
1,
33-52.
HUBEL,
D.
H.
(1957).
Tungsten
microelectrode
for
recording
from
single
units.
Science,
125,
549-550.
HuNSPERGER,
R.
W.
(1956).
Affektreaktionen
auf
elektrische
Reizung
im
Hirnstamm
der
Katze.
Helv.
physiol.
acta,
14,
70-92.
INGVAR,
D.
H.
&
HUNTER,
J.
(1953).
Influence
of
visual
cortex
on
light
impulses
in
the
brain
stem
of
the
anaesthetised
cat.
Acta
physiol.
scand.
33,
194-218.
JACKSON,
J.
H.
(1898).
Remarks
on
the
relations
of
different
divisions
of
the
central
nervous
system
to
one
another
and
to
parts
of
the
body.
Brit.
med.
J.
i,
65-69.
KELLER,
A.
D.
(1932).
Autonomic
discharges
elicited
by
physiological
stimuli
in
mid-brain
preparations.
Amer.
J.
Physiol.
100,
576-586.
KLUVER,
H.
&
BARRERA,
E.
(1953).
A
method
for
the
combined
staining
of
cells
and
fibres
in
the
nervous
system.
J.
Neuropath,
12,
400-403.
LINDSLEY,
D.
B.,
BOWDEN,
J.
&
MAGOUN,
H.
W.
(1949).
Effect
upon
the
EEG
of
acute
injury
to
the
brain
stem
activating
system.
Electroenceph.
cdin.
Neurophysiol.
1,
475-486.
MAGOUN,
H.
W.
(1958).
The
Waking
Brain,
Springfield:
Thomas.
RANDT,
C.
T.,
COLLINS,
W.
F.,
DAVIS,
H.
S.
&
DILLON,
W.
N.
(1958).
Differential
suscepti-
bility
of
afferent
pathways
to
anaesthetic
agents
in
the
cat.
Amer.
J.
Physiol.
192,
305-310.
SCHEIBEL,
M.,
SCHEIBEL,
A.,
MOLLICA,
A.
&
MoRuzzI,
G.
(1955).
Convergence
and
inter-
action
of
afferent
impulses
on
single
units
of
reticular
formation.
J.
Neurophysiol.
18,
309-331.
SPRAGUE,
J.
M.,
CHAMBERS,
W.
W.
&
STELLAR,
E.
(1961).
Attentive,
affective
and
adaptive
behaviour
in
the
cat.
Science,
133,
165-173.
STARZL,
T.
E.,
TAYLOR,
C.
W.
&
MAGOUN,
H.
W.
(1951a).
Ascending
conduction
in
reticular
activating
system,
with
special
reference
to
the
diencephalon.
J.
Neurophysiol.
14,
461-477.
STARZL,
T.
E.,
TAYLOR,
C.
W.
&
MAGOUN,
H.
W.
(1951b).
Collateral
afferent
excitation
of
reticular
formation
of
brain
stem.
J.
Neurophysiol.
14,
479-496.
WOODWORTH,
R.
S.
&
SHERRINGTON,
C.
S.
(1904).
A
pseudaffective
reflex
and
its
spinal
path.
J.
Physiol.
31,
234-243.